A Quantum Canada For All
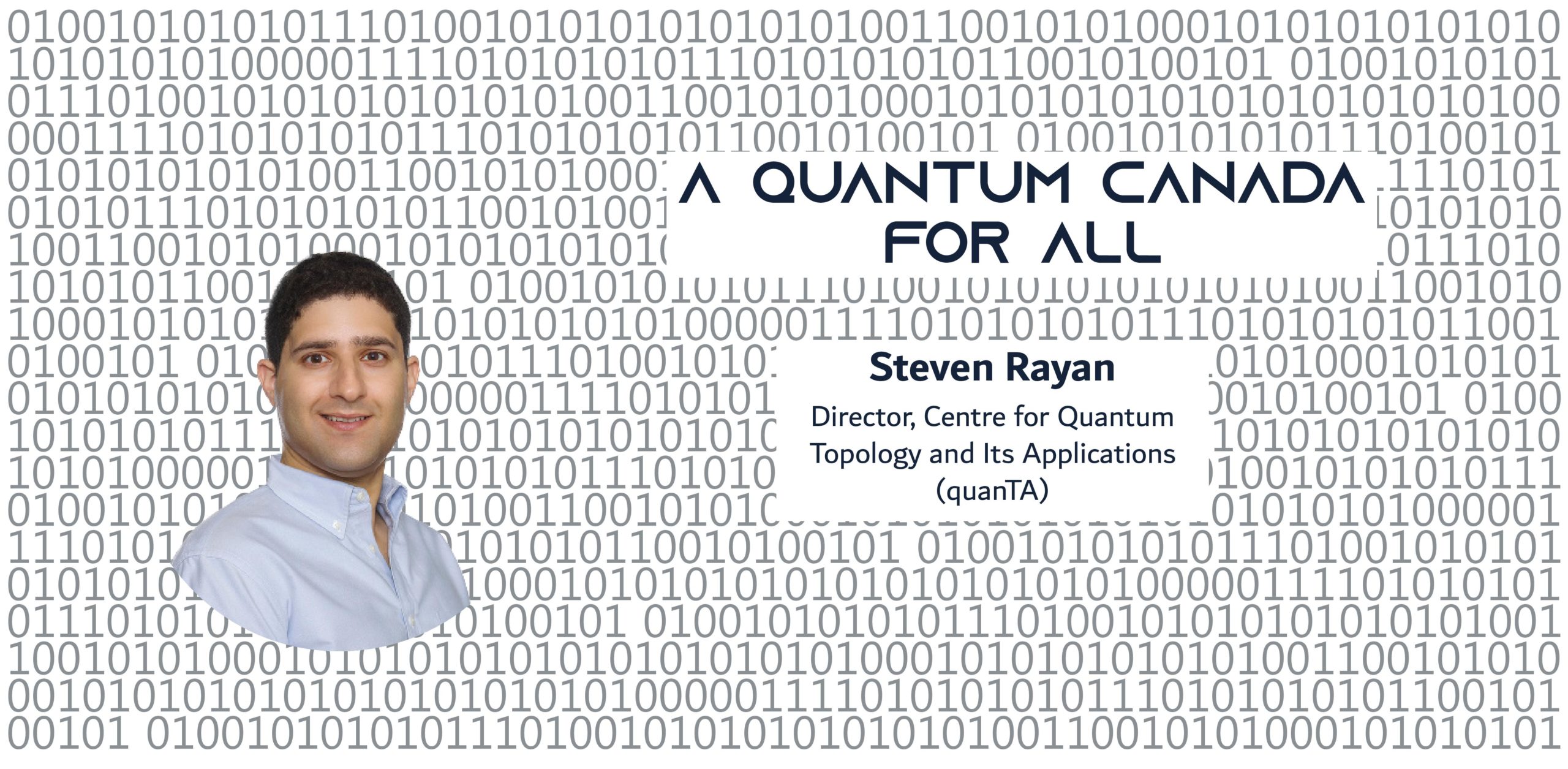
Author(s):
Steven Rayan
Centre for Quantum Topology and Its Applications (quanTA)
Director
University of Saskatchewan
Associate Professor, Mathematics & Statistics
While Covid-19 has laid waste to the best-intentioned plans of 2020, the “19” in its name reminds us that this troublesome virus originated some time in 2019. Just two months prior to the first reported cases of the novel coronavirus was a major scientific announcement, all but drowned out now by the constant hum of pandemic-related news. That announcement was of Quantum Supremacy, a feat claimed by Google on their Sycamore quantum computer.
The breakthrough has raised many questions in the general public. Obviously, what is “Quantum Supremacy”? Another question concerns the existence of quantum computers themselves. What are they? Are they “real”?
I will hold off on entertaining these questions for a moment. There’s nothing wrong with a little suspense. At the same time, this allows me the opportunity to set the stage in a different way, by focusing on a few historical scientific developments that led us here. By the end of this note, I will reflect on Canada’s contributions to the grand challenge of quantum innovation and how we can maintain a leading edge.
In the Beginning, There Was Quantum Mechanics
Quantum mechanics is a tour de force chapter in the history of science. In a period of less than 30 years in the first half of the 20th Century, physicists went from the first inklings of a new theory to a robust and well fleshed-out system of mathematical rules through which we now view the behaviour of matter at the tiniest of length scales. Yet, the famed physicist Richard Feynman still quipped, “I think I can safely say that nobody really understands quantum mechanics.” That hasn’t stopped anyone from using it, thankfully. I like to interpret his comment in the most positive way possible: that quantum science has many more gifts to give us than we can possibly predict.
Like all good science, quantum mechanics was a solution to a problem. New and better laboratory devices in the late 19th and early 20th centuries paved the way for new experiments, ones that could peer for the first time at the inner structure of matter. The results of some of these experiments defied common scientific sense. Surely a beam of electrons fired squarely at a strip of solid material between two closely-spaced openings will be repelled by the material and not cross to the other side. Experiments revealed the opposite, however, with the beam resolving on a wall on the other side. What must be is that electrons behave not only as particles but also as waves, which can disperse and send their energy through the slits. If subatomic matter and waves are indistinguishable, then that means that the position of a subatomic particle is just a
probability rather than a certainty. With such considerations in mind, quantum mechanics was born.
Has quantum mechanics affected our everyday lives? Certainly. What one might dub “Quantum 1.0” is the first wave of technologies influenced by these ideas. Quantum 1.0 is all around us, operating in plain sight for decades. Its devices include not only basic components such as transistors, but also life-saving inventions such as MRI machines, which depend crucially on the quantum-mechanical property of spin.
Writing Quantum 1.0 comes with the obvious promise of Quantum 2.0, which is what this article is really about. Just as deciding when such eras begin and end is a somewhat arbitrary game, so is deciding which technologies are definitely part of 2.0 and not 1.0. I would like to take a stance here, cutting through the noise of so much innovation to isolate two things that I believe are setting Quantum 2.0 in motion: quantum computing and quantum materials.
Quantum Computing
We need to talk about this one first. If there is a poster child for the nascent quantum revolution, it is the quantum computer. It is an awesome feat of engineering and perhaps one of the most daring things that humanity has set its collective mind to: control the fates of individual, probabilistic electrons enough to produce reasonable outcomes interpretable as “answers” to calculations, yet at the same time take advantage of their inherently unpredictable nature to explore a vast range of possible outcomes all at once.
A traditional computer is based on switches that occupy an “off” or “on” state, equivalently a “0” or “1”, at any given moment. Such a switch might arise in a simple way from a voltage differential (above a certain threshold, the value is “1”) or be realized as an integrated “flip-flop” circuit that can be forced into one state or another, which in and of itself is a Quantum 1.0 device. These circuits are valuable because we have an extremely high degree of confidence about what the state is at a given moment. Below its surface, though, are building blocks of matter that have a switch-like nature of their own — for instance, an electron with spin up or spin down — but which are subject to the uncertainty of quantum mechanics. We might say that the electron is occupying both states simultaneously with some probability for each.
Controlling an individual electron is incredibly hard work, requiring an experimental setup that is super-cooled to temperatures just barely above absolute zero. Why try to turn one into a switch when we have macroscopic circuits that work perfectly well? Why trade confidence for uncertainty? From a computational point of view, an electron in a
superposition of two states is akin to saying that two calculations can happen at once — the calculation that would result from picking 0 and the one that would result from picking 1. If you manage to harness two electrons, then you can perform 4 calculations at once (00, 01, 10, and 11). And so it goes like this, exponentially.
Given that classical switches are called bits, the custom is to call their quantum counterparts qubits. There are various models for physical realizations of qubits, including superconducting qubits such as the transmon type found in IBM’s “IBM Q” quantum computers — and yes, quantum computers are here and they are real, even if they are still considered prototypes.
The speed-up possible with qubits is important for any problem in which we must select the best outcome from a vast expanse of possibilities. One such problem is factoring an extremely large integer into its prime factors. This is exactly what Google’s 53-qubit quantum computer Sycamore achieved in 2019. This has immense implications for computer security, which currently depends on our inability to factor such primes rapidly on classical computers. What would take even the most powerful classical computers 10,000 years to do, Sycamore was able to do in just over 3 minutes: this is Quantum Supremacy.
While the feat leads to well-justified fears regarding “quantum attacks” against which our current standards of cryptography have no chance, we are not yet living in a world where the average hacker has a cryogenically-cooled array of 50 or more qubits sitting in their basement. In the meantime, we can consider other problems for which quantum computing is ideally suited. One that feels very close to home at the moment is vaccine development. At the heart of this problem is protein folding. An individual protein consists of an arrangement of amino acids bound together. One wants to determine the configuration that minimizes the energy of the protein, which is its so-called “native structure”. Given the vast number of possible configurations in three-dimensional space (a protein chain of 100 amino acids has roughly 10^47 configurations), the problem is a classical nightmare. But this is exactly the kind of problem that quantum computers find easy. Given that protein structures play a central role in antigen and vaccine design, it would seem that quantum computers have a role to play in resolving pandemics quickly.
Quantum Materials
Quantum computers need quantum ingredients. In particular, the production of easily deployable and commercially-viable quantum computing technology will require materials with robust physical properties, such as electrical conductivity with little to no variance despite extreme conditions of operations. There exist novel phases of matter, the so-called topological materials, that behave in this manner and whose theoretical
prediction was awarded a Nobel Prize in Physics in 2016. Today, hundreds of examples of these materials are known. The “topological” in their name is a nod to the mathematics behind the scenes in this amazing discovery. Topology is the branch of mathematics concerned with geometric properties that are preserved when we stretch and deform objects. The now-mandatory illustration of topology is that, to a topologist’s eyes, there is no difference between a donut and a coffee mug. They share an essential topological feature, which is the donut hole (equivalently, the hole in the mug’s handle).
How and why does topology enter the world of conductivity? It is reasonable to model conductivity as the hopping of electrons from one site to another in the microscopic, crystalline structure of a material, especially at the super-cool temperatures involved in quantum computing. These electrons and the quantum-mechanical equations that govern them give rise to a geometric surface, made up of all of the allowable energy levels that the electrons may occupy. It turns out that, for some materials, the topology of this surface controls the conductivity of the overall material. As long as the topology is protected — that is, as long as we don’t tear any holes in this quantum surface — the conductivity is robust and perfectly controlled. Most defects in the outward physical engineering of the material have no effect here, making these materials incredibly desirable for sensitive applications. (Incidentally, the Nobel Prize Committee attempted to demonstrate this on stage by tearing apart pastries.)
Topological materials are just one example of a wider class of quantum materials with novel behaviours emerging from genuinely quantum effects within them. These materials are quickly leading to new models of data storage and medical imaging devices, in addition to providing new possibilities for cultivating stable qubits.
Building a Quantum Canada
With so much happening in quantum technology, we ought to ask ourselves: how much of it is happening here in Canada?
The answer is quite a lot. Canada is home to an ever-growing number of established firms, start-ups, and research institutes all with a primary focus on quantum science or quantum technology development. Compiling an exhaustive list is not easy and one is bound to leave out important names. Sticking therefore with just a few, it would be impossible to not mention the Burnaby, BC-based D-Wave Systems, the world’s first company to manufacture commercial quantum computers. With the rise of quantum computing has come a need for quantum programming. Quantum software companies such as Vancouver’s 1QBit are leading the pack here. On the academic side, Canada enjoys the presence of the Institute for Quantum Computing at the University of Waterloo, the Institute for Quantum Science and Technology at the University of Calgary,
and the very new Quantum Algorithms Institute at Simon Fraser University, as well as several other institutes and centres centred around quantum technology, computing, and information. The Stuart Blusson Quantum Matter Institute at the University of British Columbia represents Canada’s leadership in the development of quantum materials, and there are other bright sparks on the horizon due to the Pacific Institute for Mathematical Sciences’ recent investment in quantum topology in the Prairie provinces.
This flurry of activity makes Canada a definite contender for the title of “quantum country”. How will we maintain momentum in this direction and prevent stagnancy as other countries ramp up their quantum infrastructure? Here are two thoughts:
- Interactions between Industry and Academia
First of all, these interactions need to exist. Once they are in motion, they need to be authentic, substantial, and sustained. These collaborations will be the fuel for widespread quantum innovation in Canada. The problem with fostering these interactions is a fundamental incompatibility in the way these spheres operate. Companies are looking for products — better yet, ones that people will purchase. Academics are looking for publishable results — better yet, ones that people will cite. These types of collaborations need to be incentivized, especially within academia. Academics working with quantum companies need these collaborations to be on par with publishing, as far as tenure and promotion are concerned.
This is not to say that these interactions are not occurring. They certainly are. But they can be happening much more often and much more routinely. Shared infrastructure is another catalyst for these interactions. An excellent model is the recently-established Chicago Quantum Exchange, a partnership between four universities, two government laboratories, and a number of industry partners, involving over 100 researchers at the time of writing. There is no reason why this model cannot be implemented in multiple regions in Canada.
- Quantum Training
It should go without saying that quantum technology development requires quantum ready workers. Who staffs today’s quantum companies? These firms attract highly qualified individuals: mathematicians, physicists, chemists, computer scientists, electrical and nanoscale engineers, biologists. A great many of these workers possess PhDs, obtained in Canada or internationally. If they are lucky, they may have had direct exposure to quantum computing or other quantum technologies during their studies. Many will not have, though. Many of them will enter the industrial job market after their terminal degree or a postdoctoral fellowship and are hired into tech start-ups by open-minded recruiters and project managers, often after some retraining through a Python or machine learning bootcamp, etc. Even after being hired, there is likely much more retraining involved — after all, quantum computing is as much about refrigeration as it is about math and quantum mechanics.
There was a time not too long ago when there was no such thing as a degree in computer science. It is fair to say that, in most cases, the emergence of classical computer technology preceded the existence of computer science programs. Many such programs were spun out of mathematics and engineering programs (and today dwarf mathematics programs and rival engineering programs for students).
Might programs of study focused around quantum computing or quantum technology begin to find a footing of their own? I can imagine cries that one cannot or should not learn about anything quantum without a solid grounding in other disciplines first or that an undergraduate degree around quantum technology is either too ill-defined or too focused, paradoxically. I bet there were similar protestations around the emerging computer science programs some time ago.
This provides yet another opportunity for me to take a stance: I believe such bold, new 21st Century academic programing is inevitable. In addition to producing quantum-ready workers, quantum training will introduce students to new ways of thinking that could spark innovations that we cannot yet predict, in much the same way that computer science has paved the way for computational statistics and, subsequently, artificial intelligence and machine learning.
There is no better time than to start now. While the study of quantum materials is still best approached with prior training in physics, quantum computing and the related subject of quantum information rely on firm, axiomatic principles and stand on their own as well fleshed-out subjects that can be taught from the ground up and with an interdisciplinary perspective.
Again, this is not to say that training opportunities do not exist. In Canada, the Institute for Quantum Computing offers dedicated graduate level studies in quantum computing. The newly-founded Quantum Algorithms Institute at Simon Fraser is also slated to host a quantum computing graduate program. On the US side, the Chicago Quantum Exchange is another excellent example, with undergraduate and graduate degrees in quantum information through the University of Chicago and the USA’s first master’s degree in quantum computing delivered through the University of Wisconsin-Madison. The Exchange also offers certificate programs in quantum engineering and technology. I would like to see these programs become widespread in Canada and for quantum training to begin in earnest at the undergraduate level, if not earlier. If the Ontario math curriculum has been recently updated so that students are introduced to basic ideas around coding in Grade 1 (bravo, by the way!), then why can’t they be encouraged to explore basic ideas around quantum coding in Grade 10? A quantum Canada, if it is to be an empowering agent for all, must begin in the classroom.
What is certain is that we are in the very early days of a quantum revolution, one that promises disruptive and potentially life-saving technologies. What I want to be certain about is that Canada will continue to be a leader on this front, powered by sustained academic, industry, and government collaboration, and that we will produce a generation equipped with the quantum thinking required to make the most of these innovations. I do believe that quantum thinking — akin to how it rapidly converges to the winning vaccine from a vast range of possibilities — will rapidly select for us the best and brightest future. So let’s take the bold steps necessary to empower the quantum generation in this country.